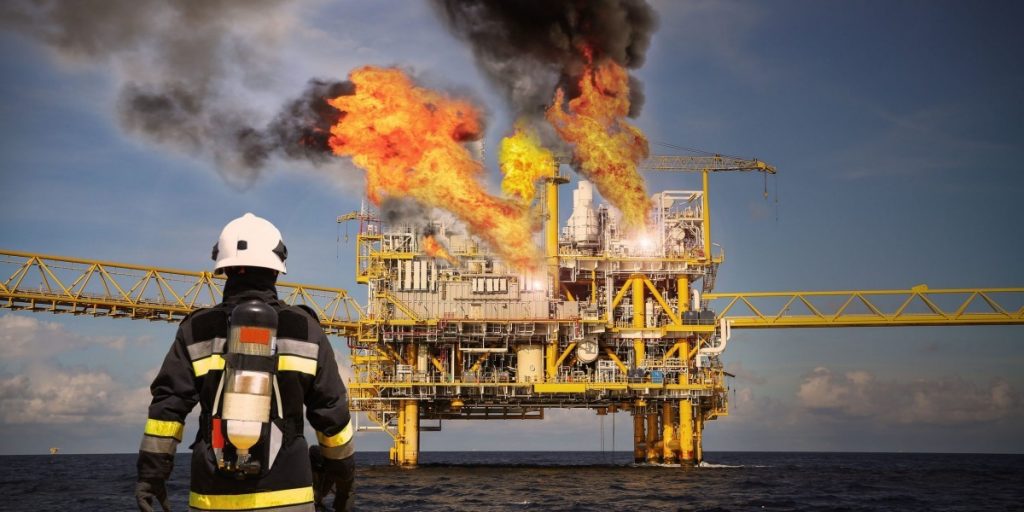
Satellite Technology for Quantifying Methane Emission and Flared gas, and Aiding Reliable Clean Energy Integration
Introduction
By monitoring Methane emissions, discerning the volume of gas flared at onshore and offshore oil and gas exploration sites, and predicting wind speeds, satellite data is increasingly useful in understanding global energy and environment systems. A major reason driving the adoption of satellite technology and data is the sheer geographic coverage they offer. For example, Landsat-8 Thermal Infrared Sensor-2 sensor is capable of scanning 185Km (115miles) of ground swath, providing a 16-day coverage cycle of the earth’s surface. However, satellites operation can also be a source of big data, placing significant demand on storage and computing resources. Take for example, Aqua—a NASA centered international Earth Science satellite mission has an average data rate of 89 Gigabytes/day. In an expected 6-year design life period, Aqua is expected to generate about 2,000 Terabyte of data.
Among the 17 sustainable development goals is the need to ensure affordable, sustainable, and clean energy for all (SDG 7) and take urgent action to combat climate change and its impact (SDG 13). If these SDG 2030 agendas are to be met ten years from now, the scale and efficiency at which data must be collected, post-processed, and distributed is pertinent. From North America to Africa, from Europe to Asia—timely environmental data is required, and satellites are perfect tools for the job.
The problem of estimating methane emission and Flared gas
According to a 2015 World Bank report, over 140 billion cubic meters of natural gas are flared annually at oil producing sites across the globe, translating to more than 350 million tons of CO2 emitted to the atmosphere. Similarly, in 2018 Environmental Protection Agency (EPA) reported that the global concentrations of methane and carbon dioxide have increased by 164 percent and 44 percent respectively from 1750 (preindustrial time) to 2016. Estimation of methane leaks is currently carried out using two methods: top-down and bottom-up approaches. The EPA utilizes the bottom-up approach in estimating methane emissions, aggregating and extrapolating onsite measured emissions from devices such as household meters to well pads, storage, distribution, and transmission facilities. The task before EPA—tallying thousands or oil and gas wells, hundreds of methane storage facilities, and over a million miles of transmission and distribution pipelines in the United States alone—is not only challenging but yields less than optimal results. In a 2018 Environmental Defense Fund paper, aircraft surveillance was used in a top-down approach to measure methane concentrations across wide geographic areas. However, air vehicle surveillance captures ambient methane from sources not associated with natural gas systems such as landfills. To avoid venting—the release of methane gas to the atmosphere during the oil and gas development process—flaring of methane is sometimes adopted. Assuming efficient and complete combustion, which is not mostly the case, flaring natural gas transforms methane emission problem to a carbon dioxide one—both of which are major contributors to global warming.
Variability and Uncertainty of Wind Energy
Wind energy is generated from the combined effects of Coriolis forces created by the earth’s rotation and the uneven heating of the earth’s surface. However, wind energy is also highly dependent on natural geographic structures such as mountains and topographic conditions such as flatness. Factors such as sea and land breezes, water and air temperatures affect wind over the ocean. All these factors act to influence and place an upper generation limit on available wind energy as a function of time—variability. Consequently, an unknown upper generation limit introduces uncertainty to power generation capacity from wind resources. From measured wind turbine data published by International Electrotechnical Commission, it can be shown that a 1 percent uncertainty in measured wind speed could result in an average of 5 percent variation in annual electricity production. Although a 1 per cent uncertainty in wind measurement may seem minor, the output implication of such measurement cannot be tolerated by investors and project developers. To optimally site wind farms, successfully bid into energy markets, reliably integrate wind power to the national grid, and adequately size investments, an efficient means of collecting wind energy data from large geographic area cannot be traded.
Satellite data for environmental protection and renewable energy improvement
Estimating the volume of flared gas
A Climate and Clean Air Commission report published in 2006 supports the argument that data on global coverage of gas flaring and its impact is limited, and when available, location of flare sites are often unreported or self-reported by companies or countries. Only a few countries maintain detailed national datasets. Given the global spread of gas flaring and methane venting locations, obtaining accurate, parallel, and independent data for verification of self-reported flaring activities is challenging, but yet necessary. With capacity to collect data over large geographic regions, remote sensing satellite data have been deployed for estimating and verifying reported volumes of gas flared by companies and countries. However, it is important to note that no satellite data is currently optimized for direct detection of gas flares and estimation of flared gas volume. Perhaps the most notable satellite sensor employed for collecting flared gas images is the National Oceanic and Atmospheric Administration’s (NOAA) Visual and Infrared Radiometer Suite (VIIRS) of detectors. Launched onboard SUOMI NPP in 2011, VIIR sensor is capable of scanning a 3,000km ground stripe at 400m resolution in 22 spectral bands coverage from 412nm to 12um. VIIRS is can to discriminate various hot sources, using intensity of captured light at night to estimate gas flaring rate at oil and gas facilities across the globe. For example, it can exclude non-flare hot sources from biomass burning sources by filtering off emissions with temperature below 1400 deg K. Terra and Aqua satellites both flew MODIS (Moderate Resolution Imaging Spectrometer) instruments. MODIS uses its 36 discrete spectral bands to collect a variety of Earth’s vital signs, including volcanic eruptions and wildfires. Inspired by VIIRS night fire detection work, researchers at Alberta Energy Regulator designed specialized algorithms implemented on images from Landsat-8 to allow daytime detection of gas flares regardless of solar reflectance.
Note: Image of the area spanning across Kuwait, Iraq, and Iran. (Left) DMSP detections include both gas flares and light from towns and cities. (Right) Image from VIIRS detects gas flares exclusively, discriminating lights from surrounding towns and cities.
Source: World Bank’s Global Gas Flaring Reduction Partnership: Gas Flaring Estimates, Methodology for determining the flare volumes from satellite data. http://pubdocs.worldbank.org/en/853661587048977000/Estimation-of-flare-gas-volumes-from-satellite-data-002.pdf
Measuring methane emission rate
While ground-based sensors are one of the most accurate and continuous means of estimating local methane emissions to the atmosphere, satellites offer the advantage of covering a much wider geographic area.
In 2009, the first satellite dedicated to observing Greenhouse Gases—”IBUKI” (GOSAT)—was launched. With a capacity to update data on observation points every three days, IBUKI can acquire data from as many as 56,000 observation locations worldwide—more observation locations available from ground stations or aircrafts. IBUKI does not only collect accurate data on greenhouse gases that have not been previously measured, but also provides a commonly shared criteria for the measurement of greenhouse gases. Sentinel-5 Precursor was launched in 2017 to monitor methane concentrations in the atmosphere using its sensor TROPOspheric Monitoring Instrument (TROPOMI). TROPOMI has a planned mission of seven years. However, there are other active greenhouse gas monitoring satellites or scheduled launches—GHGSat was launched in 2016. The launch of MethaneSat , TANGO, and CO2M are scheduled for 2022, 2024, and 2026 respectively.
Relationship between satellite Location and data collection
IBUKI, Sentinel-5 Precursor, GHGSat, MethaneSat, TANGO, CO2, are designed to operate in polar, sun-synchronous orbit known as low earth orbit (LEO). LEO orbits are defined as orbits between 200-1000km altitude. Satellites in LEO travel around their orbits in about 100 minutes at a speed of approximately 7.6km per second while the earth rotates underneath. Since satellites in LEO enjoy close proximity to the earth, they can potentially provide high quality images/data with moderate sensor sizes; they are cheaper and take shorter time to build. On the other hand, LEO satellites are incapable of providing continuous coverage of specific locations on surface of the earth. Conversely, satellites in Geostationary orbit are able to support uninterrupted data collection over targeted geo locations. Geostationary Carbon Observatory (GeoCarb) satellite is targeted for launch in early 2020 and is expected to make 10 million daily methane and carbon dioxide concentration observations of the Americas from a 22,236 mile (35,000Km) Geostationary orbit. Because geostationary satellites operate much further from the earth than LEO satellites, their sensors have a larger field of view. Satellites in Geostationary orbit travel at a speed equal to the Earth’s rotational speed and are continually looking over the same locations. GeoCarb, for instance, would allow a continuous “wall-to-wall” coverage of the Americas from the southern tip of Hudson Bay to southern tip of the South America.
Measurement of wind speed profile
As wind turbine rotors increase in diameter, it is increasingly becoming important to acquire wind speed profiles across a height range covering the entire rotor diameter rather than a point. SODAR (Sound Detection and Ranging) and LIDAR are two remote sensing technologies successfully applied in ground-based acquisition of vertical atmospheric wind profile between selected height intervals. SODAR uses sound propagation to probe the wind profile in the atmosphere and LIDAR (Light Detection and Ranging) uses electromagnetic radiation, microwaves, or laser light, to achieve the same.
Launched in August 2018, Aeolus satellite conducted the first Doppler wind Lidar measurement from space—testing the feasibility of direct wind profile observations for numerical weather prediction. Before the launch of Aeolus, there was no global system to measure wind speeds across the earth. Although Aeolus was built primarily to fill gaps necessary to broadly understand long term trends in climate change by observing global wind patterns in the tropic, southern Pacific, Atlantic, and over oceans, data from Aeolus can also help improve wind speed estimates for more reliable wind turbine operations. The results from Aeolus experiments are promising. After correcting for biases, Aeolus data improved wind vector estimates by up to 1.5 percent when compared with data collected from non-space sources such as radiosonde profilers and aircrafts. Validation of Aeolus wind measurement data shows that on the Mie scale, wind data were more accurate with about 1m/s systemic error. While wind turbines typically operate below 0.5 kilometer altitude, Aeolus however, can measure wind speed and direction from the Earth’s surface to an altitude of up to 30 kilometers.
Note: The charts show a reduction of up to 1.5 % in differences between radiosonde, profiler and aircraft wind observations and short-range forecasts used in the data assimilation system as a result of assimilating Aeolus data in the tropics (left) and in the extratropical southern hemisphere (right). The differences without the assimilation of Aeolus data, expressed in terms of the standard deviation, are represented by the 100% mark. The horizontal lines represent 95% confidence intervals.
Source European Centre for Medium-Range Weather Forecasts (ECMWF). Tests Show positive impact of new Aeolus wind data on forecasts (12 November 2019). https://www.ecmwf.int/en/about/media-centre/news/2019/tests-show-positive-impact-new-aeolus-wind-data-forecasts
Global and National Impacts of satellite data on Climate Policy
Global policy impacts
Under article 14 of the Paris agreement, the first periodic global stocktake of greenhouse gases is scheduled for 2023 and every 5 years thereafter. Satellites are excellent means of monitoring and quantifying GHG emissions remotely, reducing reliance on self-reporting from parties to the Paris agreement. The World Bank Global Gas Flaring Reduction (GGFR) in partnership with the United States National Oceanic and Atmospheric Administration and Colorado School of Mines developed a global gas flaring estimates based on observations from a satellite launched in 2012. Through the use of satellite data, GGFR is able to provide independent flaring data—not reliant on self-reporting. By utilizing satellite technology, an independent global stock-take of GHG emission scheduled for 2023 is feasible.
National policy impacts
Research has shown that previous economic recessions have been followed by emission rebound. An estimated 6 percent increase in greenhouse emissions from fossil-fuel related activities followed the 2008/2009 global financial in 2010. The 1970 oil crises, the U.S. savings and loans crises in the late 1980s, and the disintegration of the Soviet Union in the 1980s were all followed by ramped GHG emissions. However, scaled, more accurate, and timely availability of improved GHG emission data from satellites could serve to encourage countries to introduce stricter policies to curb methane production or imports, while facilitating emission pricing mechanisms to ultimately drive transitioning from fossil-fuel usage to clean energy. A Johns Hopkins University study argues that U.S. government COVID-19 economic recovery spending could offer a long-term climate policy pathway to decarbonization through investments in clean energy technologies that can reduce emissions. For instance, investment in satellite technologies better suited for wind speed profiling could help improve wind energy prediction, leading to optimized, more reliable integration of this intermittent energy source to the grid.
Conclusion
Anthropogenic activities of oil and gas companies across the globe that result in lethal quantities of methane emissions and gas flaring constitute a major threat to global warming. Ground-based monitoring of methane emission and gas flaring are either administered by the emitting companies or state appropriate authorities. From the perspective of global climate governance regimes such as UNFCCC, relying on self-reporting from emitting companies and countries may be misleading, leading to weak governance and policy outcomes. Data from satellite data is a reliable means to independently aggregate data across oil and gas facilities across the globe. While dedicated satellite sensors for measuring greenhouse gases have been flown or planned, there is no satellites currently existing for strictly quantifying the volume of flared gas.
Uncertainty in measured wind speed has significant effect on energy generated by wind turbines. A 1 per cent uncertainty in measured wind speed could result in an average of 5 per cent variation in annual electricity production. As wind turbine rotors increase in diameter, it is increasingly becoming essential to acquire wind speed profiles across a vertical range of interest within the atmosphere than a point in the turbine hub. SONAR and LIDAR remote technology are well suited for this purpose. Aeolus is the only satellite equipped with a LIDAR sensor dedicated to directly measure wind speed profiles . Since providing assimilated data in January 2020, Aeolus mission has shown promising outcomes. Although most suitable for climate-based research, Aeolus is, however, the first in its category to supply global wind data products. A more detailed, more accurate measurement of wind profiles is required to better support wind energy generation—Aeolus, nonetheless, has demonstrated the feasibility of direct wind profile measurement from space.
***
Reuben Umunna is a satellite engineer and graduate of the Energy, Resource and Environment program at Johns Hopkins Schools of Advanced International Studies (SAIS) (@LinkedIn)
Feature Image taken from: https://pxhere.com/en/photo/1597727